Bubbles of Brand New Stars MUSE observations and artist's impression of the HH 1177 young star system
Digitized Sky Survey image around the HII region LHA 120-N 180B
PR Image eso2318f
The HII region LHA 120-N 180B in the constellation Mensa
Videos
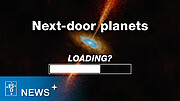
In a remarkable discovery, astronomers
have found a disc around a young star in the Large Magellanic Cloud, a
galaxy neighbouring ours. It’s the first time such a disc, identical to
those forming planets in our own Milky Way, has ever been found outside
our galaxy. The new observations reveal a massive young star, growing
and accreting matter from its surroundings and forming a rotating disc.
The detection was made using the Atacama Large Millimeter/submillimeter
Array (ALMA) in Chile, in which the European Southern Observatory (ESO)
is a partner.
“When I first saw evidence for a rotating structure in the ALMA data I could not believe that we had detected the first extragalactic accretion disc, it was a special moment,” says Anna McLeod, an associate professor at Durham University in the UK and lead author of the study published today in Nature. “We know discs are vital to forming stars and planets in our galaxy, and here, for the first time, we’re seeing direct evidence for this in another galaxy.”
This study follows up observations with the Multi Unit Spectroscopic Explorer (MUSE) instrument on ESO’s Very Large Telescope (VLT), which spotted a jet from a forming star — the system was named HH 1177 — deep inside a gas cloud in the Large Magellanic Cloud. “We discovered a jet being launched from this young massive star, and its presence is a signpost for ongoing disc accretion,” McLeod says. But to confirm that such a disc was indeed present, the team needed to measure the movement of the dense gas around the star.
As matter is pulled towards a growing star, it cannot fall directly onto it; instead, it flattens into a spinning disc around the star. Closer to the centre, the disc rotates faster, and this difference in speed is the smoking gun that shows astronomers an accretion disc is present.
“The frequency of light changes depending on how fast the gas emitting the light is moving towards or away from us,” explains Jonathan Henshaw, a research fellow at Liverpool John Moores University in the UK, and co-author of the study. “This is precisely the same phenomenon that occurs when the pitch of an ambulance siren changes as it passes you and the frequency of the sound goes from higher to lower.”
The detailed frequency measurements from ALMA allowed the authors to distinguish the characteristic spin of a disc, confirming the detection of the first disc around an extragalactic young star.
Massive stars, like the one observed here, form much more quickly and live far shorter lives than low-mass stars like our Sun. In our galaxy, these massive stars are notoriously challenging to observe and are often obscured from view by the dusty material from which they form at the time a disc is shaping around them. However, in the Large Magellanic Cloud, a galaxy 160 000 light-years away, the material from which new stars are being born is fundamentally different from that in the Milky Way. Thanks to the lower dust content, HH 1177 is no longer cloaked in its natal cocoon, offering astronomers an unobstructed, if far away, view of star and planet formation.
“We are in an era of rapid technological advancement when it comes to astronomical facilities,” McLeod says. “Being able to study how stars form at such incredible distances and in a different galaxy is very exciting.”
“When I first saw evidence for a rotating structure in the ALMA data I could not believe that we had detected the first extragalactic accretion disc, it was a special moment,” says Anna McLeod, an associate professor at Durham University in the UK and lead author of the study published today in Nature. “We know discs are vital to forming stars and planets in our galaxy, and here, for the first time, we’re seeing direct evidence for this in another galaxy.”
This study follows up observations with the Multi Unit Spectroscopic Explorer (MUSE) instrument on ESO’s Very Large Telescope (VLT), which spotted a jet from a forming star — the system was named HH 1177 — deep inside a gas cloud in the Large Magellanic Cloud. “We discovered a jet being launched from this young massive star, and its presence is a signpost for ongoing disc accretion,” McLeod says. But to confirm that such a disc was indeed present, the team needed to measure the movement of the dense gas around the star.
As matter is pulled towards a growing star, it cannot fall directly onto it; instead, it flattens into a spinning disc around the star. Closer to the centre, the disc rotates faster, and this difference in speed is the smoking gun that shows astronomers an accretion disc is present.
“The frequency of light changes depending on how fast the gas emitting the light is moving towards or away from us,” explains Jonathan Henshaw, a research fellow at Liverpool John Moores University in the UK, and co-author of the study. “This is precisely the same phenomenon that occurs when the pitch of an ambulance siren changes as it passes you and the frequency of the sound goes from higher to lower.”
The detailed frequency measurements from ALMA allowed the authors to distinguish the characteristic spin of a disc, confirming the detection of the first disc around an extragalactic young star.
Massive stars, like the one observed here, form much more quickly and live far shorter lives than low-mass stars like our Sun. In our galaxy, these massive stars are notoriously challenging to observe and are often obscured from view by the dusty material from which they form at the time a disc is shaping around them. However, in the Large Magellanic Cloud, a galaxy 160 000 light-years away, the material from which new stars are being born is fundamentally different from that in the Milky Way. Thanks to the lower dust content, HH 1177 is no longer cloaked in its natal cocoon, offering astronomers an unobstructed, if far away, view of star and planet formation.
“We are in an era of rapid technological advancement when it comes to astronomical facilities,” McLeod says. “Being able to study how stars form at such incredible distances and in a different galaxy is very exciting.”
Source: ESO/News
More information
This research was presented in a paper titled “A likely Keplerian disk
feeding an optically revealed massive young star” to appear in Nature (doi: 10.1038/s41586-023-06790-2 ; url: https://www.nature.com/articles/s41586-023-06790-2). The disk was spotted within a region in the Large Magellanic Cloud known as LHA 120-N 180B, which had been featured in a previous ESO press release, titled "Bubbles of Brand New Stars".
The team is composed of A. F. McLeod (Centre for Extragalactic Astronomy, Department of Physics, Durham University, UK; Institute for Computational Cosmology, Department of Physics, University of Durham, UK), P. D. Klaassen (UK Astronomy Technology Centre, Royal Observatory Edinburgh, UK), M. Reiter (Department of Physics and Astronomy, Rice University, USA), J. Henshaw (Astrophysics Research Institute, Liverpool John Moores University, UK; Max Planck Institute for Astronomy, Germany), R. Kuiper (Faculty of Physics, University of Duisburg-Essen, Germany), and A. Ginsburg (Department of Astronomy, University of Florida, USA).
The Atacama Large Millimeter/submillimeter Array (ALMA), an international astronomy facility, is a partnership of ESO, the U.S. National Science Foundation (NSF) and the National Institutes of Natural Sciences (NINS) of Japan in cooperation with the Republic of Chile. ALMA is funded by ESO on behalf of its Member States, by NSF in cooperation with the National Research Council of Canada (NRC) and the National Science and Technology Council (NSTC) in Taiwan and by NINS in cooperation with the Academia Sinica (AS) in Taiwan and the Korea Astronomy and Space Science Institute (KASI). ALMA construction and operations are led by ESO on behalf of its Member States; by the National Radio Astronomy Observatory (NRAO), managed by Associated Universities, Inc. (AUI), on behalf of North America; and by the National Astronomical Observatory of Japan (NAOJ) on behalf of East Asia. The Joint ALMA Observatory (JAO) provides the unified leadership and management of the construction, commissioning and operation of ALMA.
The European Southern Observatory (ESO) enables scientists worldwide to discover the secrets of the Universe for the benefit of all. We design, build and operate world-class observatories on the ground — which astronomers use to tackle exciting questions and spread the fascination of astronomy — and promote international collaboration for astronomy. Established as an intergovernmental organisation in 1962, today ESO is supported by 16 Member States (Austria, Belgium, the Czech Republic, Denmark, France, Finland, Germany, Ireland, Italy, the Netherlands, Poland, Portugal, Spain, Sweden, Switzerland and the United Kingdom), along with the host state of Chile and with Australia as a Strategic Partner. ESO’s headquarters and its visitor centre and planetarium, the ESO Supernova, are located close to Munich in Germany, while the Chilean Atacama Desert, a marvellous place with unique conditions to observe the sky, hosts our telescopes. ESO operates three observing sites: La Silla, Paranal and Chajnantor. At Paranal, ESO operates the Very Large Telescope and its Very Large Telescope Interferometer, as well as survey telescopes such as VISTA. Also at Paranal ESO will host and operate the Cherenkov Telescope Array South, the world’s largest and most sensitive gamma-ray observatory. Together with international partners, ESO operates ALMA on Chajnantor, a facility that observes the skies in the millimetre and submillimetre range. At Cerro Armazones, near Paranal, we are building “the world’s biggest eye on the sky” — ESO’s Extremely Large Telescope. From our offices in Santiago, Chile we support our operations in the country and engage with Chilean partners and society.
The team is composed of A. F. McLeod (Centre for Extragalactic Astronomy, Department of Physics, Durham University, UK; Institute for Computational Cosmology, Department of Physics, University of Durham, UK), P. D. Klaassen (UK Astronomy Technology Centre, Royal Observatory Edinburgh, UK), M. Reiter (Department of Physics and Astronomy, Rice University, USA), J. Henshaw (Astrophysics Research Institute, Liverpool John Moores University, UK; Max Planck Institute for Astronomy, Germany), R. Kuiper (Faculty of Physics, University of Duisburg-Essen, Germany), and A. Ginsburg (Department of Astronomy, University of Florida, USA).
The Atacama Large Millimeter/submillimeter Array (ALMA), an international astronomy facility, is a partnership of ESO, the U.S. National Science Foundation (NSF) and the National Institutes of Natural Sciences (NINS) of Japan in cooperation with the Republic of Chile. ALMA is funded by ESO on behalf of its Member States, by NSF in cooperation with the National Research Council of Canada (NRC) and the National Science and Technology Council (NSTC) in Taiwan and by NINS in cooperation with the Academia Sinica (AS) in Taiwan and the Korea Astronomy and Space Science Institute (KASI). ALMA construction and operations are led by ESO on behalf of its Member States; by the National Radio Astronomy Observatory (NRAO), managed by Associated Universities, Inc. (AUI), on behalf of North America; and by the National Astronomical Observatory of Japan (NAOJ) on behalf of East Asia. The Joint ALMA Observatory (JAO) provides the unified leadership and management of the construction, commissioning and operation of ALMA.
The European Southern Observatory (ESO) enables scientists worldwide to discover the secrets of the Universe for the benefit of all. We design, build and operate world-class observatories on the ground — which astronomers use to tackle exciting questions and spread the fascination of astronomy — and promote international collaboration for astronomy. Established as an intergovernmental organisation in 1962, today ESO is supported by 16 Member States (Austria, Belgium, the Czech Republic, Denmark, France, Finland, Germany, Ireland, Italy, the Netherlands, Poland, Portugal, Spain, Sweden, Switzerland and the United Kingdom), along with the host state of Chile and with Australia as a Strategic Partner. ESO’s headquarters and its visitor centre and planetarium, the ESO Supernova, are located close to Munich in Germany, while the Chilean Atacama Desert, a marvellous place with unique conditions to observe the sky, hosts our telescopes. ESO operates three observing sites: La Silla, Paranal and Chajnantor. At Paranal, ESO operates the Very Large Telescope and its Very Large Telescope Interferometer, as well as survey telescopes such as VISTA. Also at Paranal ESO will host and operate the Cherenkov Telescope Array South, the world’s largest and most sensitive gamma-ray observatory. Together with international partners, ESO operates ALMA on Chajnantor, a facility that observes the skies in the millimetre and submillimetre range. At Cerro Armazones, near Paranal, we are building “the world’s biggest eye on the sky” — ESO’s Extremely Large Telescope. From our offices in Santiago, Chile we support our operations in the country and engage with Chilean partners and society.
Links
- Research paper
- Photos of ALMA
- For journalists: subscribe to receive our releases under embargo in your language
- For scientists: got a story? Pitch your research
Contacts
Anna McLeod
Centre for Extragalactic Astronomy, Durham University
Durham, UK
Tel: +44 191 334 3789
Email: anna.mcleod@durham.ac.uk
Jonathan Henshaw
Astrophysics Research Institute, Liverpool John Moores University
Liverpool, UK
Email: J.D.Henshaw@ljmu.ac.uk
Bárbara Ferreira
ESO Media Manager
Garching bei München, Germany
Tel: +49 89 3200 6670
Cell: +49 151 241 664 00
Email: press@eso.org
Anna McLeod
Centre for Extragalactic Astronomy, Durham University
Durham, UK
Tel: +44 191 334 3789
Email: anna.mcleod@durham.ac.uk
Jonathan Henshaw
Astrophysics Research Institute, Liverpool John Moores University
Liverpool, UK
Email: J.D.Henshaw@ljmu.ac.uk
Bárbara Ferreira
ESO Media Manager
Garching bei München, Germany
Tel: +49 89 3200 6670
Cell: +49 151 241 664 00
Email: press@eso.org